Photonic Optical Fibers Laboratory
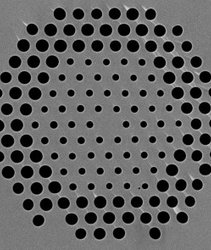
Photonic Optical Fibers Laboratory
Head of the laboratory: | dr hab. Rafał Kasztelanic |
Localisation: | ul. Pasteura 7, 0.15 |
Institute: | IG |
Department: | PD |
The Photonic Optical Fibers Laboratory focus is the design, modeling, fabrication, characterization, and use of all kinds of optical fibers. In particular, research is carried out towards the use of nonlinear phenomena in optical fibers to generate the supercontinuum spectrum, i.e. white laser. Work is also underway on amplifiers, fiber optic switches, and femtosecond pulses. Photonic optical fibers filled with liquids are also tested.
Research area
Classic optical fibers used e.g. in telecommunications, conduct light thanks to the phenomenon of total internal reflection. It consists in the fact that the light falling at a large angle on the border between two materials from the side of the material with a higher refractive index does not pass to the material with a lower refractive index but is reflected from the border and remains in the first material. Therefore, the light coupling into the core of an optical fiber made of a material with a higher refractive index gets trapped therein. Such optical fibers, thanks to their high bandwidth and low losses, are very well suited for data transmission. However, they are not free from weaknesses and all kinds of limitations. One of the main disadvantages is chromatic dispersion. This phenomenon is responsible for the slower propagation of light with a shorter wavelength. Consequently, it leads to a time-stretching of the transmitted signal and a reduction in the achievable transmission rate. Another disadvantage is the limitation of the amount of energy that can be transmitted through the optical fiber. The disadvantage is, on the one hand, the occurrence of small non-linear effects and, on the other hand, difficulties in achieving high non-linear effects.
Many different types of optical fibers have been developed searching solutions to the above-mentioned problems. One approach is to modify the optical fiber by adding air holes. Such optical fibers can be generally called photonic optical fibers. We can distinguish several types of them. In optical fibers with a full core and the structure of openings around the core, the light-guiding mechanism is similar to that in classic fibers. The core has a higher refractive index and there are air holes with a lower refractive index around the core. Such optical fibers allow for dispersion control. That is why, among others, the usage for dispersion compensation in telecommunications fibers.
It turns out, however, that similar-looking optical fibers can also be made with a hollow core. In this case, the total internal reflection mechanism will not work because the refractive index in the core is smaller than around it. However, the structure of the holes can be designed in such a way as to obtain a so-called photonic band-gap for certain wavelength intervals. This band-gap "forbids" the light from leaving the core through the structure of the holes to the outside. And since light cannot escape, it propagates through the core. Such optical fibers are used, among others, for transmitting high energy light as there is no heating of the fiber material.
There is another mechanism used in optical fibers to guiding light. In so-called anti-resonance fibers, light is reflected from the smooth capillaries surrounding the core. For certain wavelengths, there is a strong resonance between the waves reflected from different surfaces, resulting in high fiber losses. However, between these wavelengths, there are ranges where such resonances do not occur and light propagates with very little loss with a large hollow core.
Fig. 1 Examples of photonic fibers: a) all-glass fiber with a nano-structured parabolic core, b) full-core photonic fiber, c) hollow-core photonic fiber, d) anti-resonance fiber.
Photonic optical fibers allow, on the one hand, the efficient use of non-linear effects and at the same time the dispersion control. Both of these factors are used to produce the supercontinuum spectrum. It turns out that the sequence of short coherent light pulses (femtosecond pulses) introduced into the appropriate optical fiber is stretched by non-linear interactions. As a result, at the output of such a fiber, a broadening light spectrum is obtained, e.g. in the entire range of visible light.
Fig. 2 Source of the supercontinuum: a) photonic optical fiber to generate the supercontinuum spectrum, b) experimentally generated spectrum, c) simulation result of the pulse broadening.
In order to increase the efficiency of inducing nonlinear effects, which may translate into a wider spectrum or the possibility of building smaller devices, our Laboratory examines the behavior of photonic optical fibers filled with liquids characterized by high non-linearity.
Fig. 3 Liquid-filled photonic fiber for supercontinuum spectra generation: a) empty optical fiber, b) fiber core filled with carbon tetrachloride, c) experimental setup, d) spectral broadening simulation result, e) experimental result.
Publications
X. Forestier, T. Karpate, G. Huss, V. Tombelaine, G. Stępniewski, A. Anuszkiewicz, R. Kasztelanic, A. Filipkowski, D. Pysz, M. Klimczak, R. Buczyński, Supercontinuum generation in nanostructured core gradient index fibers, Appl. Nanosci., 10, 1997–2005 (2020).
M. Longobucco, I. Astrauskas, A. Pugžlys, D. Pysz, F. Uherek, A. Baltuška, R. Buczyński, I. Bugár, Broadband Self-Switching of Femtosecond Solitons in Highly Nonlinear High Index Contrast Dual-Core Fibre, Opt. Comm., 472, 126043 (2020).
T. Karpate, G. Stepniewski, D. Pysz, A. Rampur, Y. Stiepanienko, R. Buczynski, M. Klimczak, Soliton detuning of 68.5 THz in the near-infrared in a highly nonlinear suspended core tellurite fiber, JOSA B, 37(5), 1502-1509 (2020).
V.T. Hoang, R. Kasztelanic, G. Stepniewski, K.D. Xuan, V.C. Long, M. Trippenbach, R M. Klimczak, Buczyński, J. Pniewski, Femtosecond supercontinuum generation around 1560 nm in hollow-core photonic crystal fibers filled with carbon tetrachloride, Appl. Opt. 59(12), 3720-3725 (2020).
L.C. Van, V.T. Hoang, V.C. Long, K. Borzycki, K.D. Xuan, V. T. Quoc, M. Trippenbach, R. Buczyński, J. Pniewski, Supercontinuum generation in photonic crystal fibers infiltrated with nitrobenzene, Las. Phys. 30(3), 035105 (2020).
M. Longobucco, P. Stajanča, L. Čurilla, R. Buczyński, I. Bugár, Applicable ultrafast all-optical switching by soliton self-trapping in high index contrast dual-core fiber, Laser Phys. Lett., 17, 025102 (2020).
D. Dobrakowski, A. Rampur, G. Stepniewski, D. Pysz, L. Zhao, Y. Stiepanienko, R. Buczynski, M. Klimczak, Femtosecond pulse delivery around 1560 nm in large core inhibited coupling fibers, JOSA B, 36(11), 3030-3038 (2019).
M. Klimczak, D. Dobrakowski, A.N. Ghosh, G. Stepniewski, D. Pysz, G. Huss, T. Sylvestre, R. Buczynski, Nested-capillary anti-resonant silica fiber with mid-infrared transmission and low bending sensitivity at 4000 nm, Opt. Lett., 44(17) 4395-4398 (2019).
L.C. Van, V.T. Hoang, V.C. Long, K. Borzycki, K.D. Xuan, V.T. Quoc, M. Trippenbach, R. Buczyński, J. Pniewski, Optimization of optical properties of photonic crystal fibers infiltrated with chloroform for supercontinuum generation, Laser Phys. 29, 075107 (2019).
M. Longobucco, J. Cimek, L. Čurilla, D. Pysz, R. Buczynski, I. Bugar, All-optical switching based on soliton self-trapping in dual-core high-contrast optical fiber, Opt. Fib. Technol. 51, 48-58 (2019).
M. Nikodem, G. Gomolka, M. Klimczak, D. Pysz, R. Buczynski, Laser absorption spectroscopy at 2 µm inside the revolver-type anti-resonant hollow-core fiber, Opt. Express 27(10), 14998-15006 (2019).
V.T. Hoang, R. Kasztelanic, A. Filipkowski, G. Stepniewski, D. Pysz, M. Klimczak, S. Ertman, V.C. Long, T. Wolinski, M. Trippenbach, K.D. Xuan, M. Smietana, R. Buczynski, Supercontinuum generation in all-normal dispersion large core photonic crystal fiber infiltrated with carbon tetrachloride, Opt. Mat. Express 9(5). 2264-2278 (2019
M. Klimczak, D. Michalik, G. Stepniewski, T. Karpate, X. Forestier, J. Cimek, R. Kasztelanic, D. Pysz, R. Stepien, R. Buczynski, Coherent supercontinuum generation in tellurite glass regular lattice photonic crystal fibers, JOSA B, 36(2), A112-A124 (2019).
G. Stępniewski, J. Pniewski, D. Pysz, J. Cimek, R. Stępień, M. Klimczak, R. Buczyński, Development of dispersion-optimized photonic crystal fibers based on heavy metal oxide glasses for broadband infrared supercontinuum generation with fiber lasers, Sensors, 18(12), 4127 (2018).
V.T. Hoang, R. Kasztelanic, A. Anuszkiewicz,. G. Stepniewski, A. Filipkowski, S. Ertman, D. Pysz, T. Wolinski, K.D. Xuan, M. Klimczak, R. Buczynski, All-normal dispersion supercontinuum generation in photonic crystal fiber with large hollow core infiltrated with toluene, Opt. Mat. Express, 8(11), 3568-3582 (2018).
A.N. Ghosh, M. Klimczak, R. Buczynski, J.M. Dudley, T. Sylvestre, Supercontinuum Generation in Heavy-Metal Oxide Glass Based Suspended-Core Photonic Crystal Fibers, J. Opt. Soc. Am. B 35(9), 2311-2316 (2018).
T. Stefaniuk, G. Stepniewski, D. Pysz, R. Stepien, R. Buczynski, Fused silica photonic crystal fiber with heavily germanium doped microinclusion in the core dedicated to the couple, guide and control LP02 higher-order mode, Opt. Express 26(17), 21939-21949 (2018).
Q.H. Dinh, J. Pniewski, H.L. Van, A. Ramaniuk, V.C. Long, K. Borzycki, K.D. Xuan, M. Klimczak, R. Buczyński, Optimization of optical properties of photonic crystal fibers infiltrated with carbon tetrachloride for supercontinuum generation with sub-nJ femtosecond pulses, Appl. Opt., 57(14) 3738-3746 (2018).
V.T. Hoang, B. Siwicki, M. Franczyk, G. Stępniewski, H.L. Van, V.C. Long, M. Klimczak, R. Buczyński, Broadband low-dispersion low-nonlinearity photonic crystal fiber dedicated to near-infrared high-power femtosecond pulse delivery, Opt. Fib. Technol. 42, 119-125 (2018).
A. Rampur, P. Ciąćka, J. Cimek, R. Kasztelanic, R. Buczyński, M. Klimczak, Development of suspended core soft glass fibers for far-detuned parametric conversion, J. Opt. 20(4), 045501 (2018).
M. Klimczak, B. Siwicki, A. Heidt, R. Buczyński, Coherent supercontinuum generation in soft glass photonic crystal fibers, Phot. Res. 5(6), 710-727 (2017).
J. Pniewski, G. Stepniewski, R. Kasztelanic, B. Siwicki, D. Pierscinska, K. Pierscinski, D. Pysz, K. Borzycki, R. Stepien, M. Bugajski, R. Buczynski, High numerical aperture large-core photonic crystal fiber for a broadband infrared transmission, , Infrared Phys. Techn., 79, 10-16, (2016).
G. Stepniewski, R. Kasztelanic, D. Pysz, R. Stepien, M. Klimczak, R. Buczynski, Temperature sensitivity of chromatic dispersion in nonlinear silica and heavy metal oxide glass photonic crystal fibers, Opt. Mat. Express, 6(8), 2689-2703 (2016).
B. Siwicki, R. Kasztelanic, M. Klimczak, J. Cimek, D. Pysz, R. Stępień, R. Buczyński, Extending of flat normal dispersion profile in all-solid soft glass nonlinear photonic crystal fibres, J. Opt., 18, 065102, 2016.
M. Klimczak, G. Soboń, R. Kasztelanic, K.M. Abramski, R. Buczyński, Direct comparison of the shot-to-shot noise performance of all-normal dispersion and anomalous dispersion supercontinuum pumped with sub-picosecond pulse fiber-based laser, Sci. Rep., 6, 19284 (2016).
B. Siwicki, M. Klimczak, R. Stępien,, R. Buczyński, Supercontinuum Generation Enhancement in All-Solid All-Normal Dispersion Soft Glass Photonic Crystal Fiber Pumped at 1550 nm, Opt. Fib. Technol. , 25 64-71. 2015.
G. Stępniewski, J. Pniewski, M. Klimczak, T. Martynkien, D. Pysz, R. Stępień, I. Kujawa, K. Borzycki, R. Buczyński, Broadband dispersion measurement of photonic crystal fibers with nanostructured core, Opt. Quant. Electron., 47(3), 807-814 (2015).
Realted documents:
- 1. Przepisy BHP
![]() | Glovebox workstationglovebox workstation |